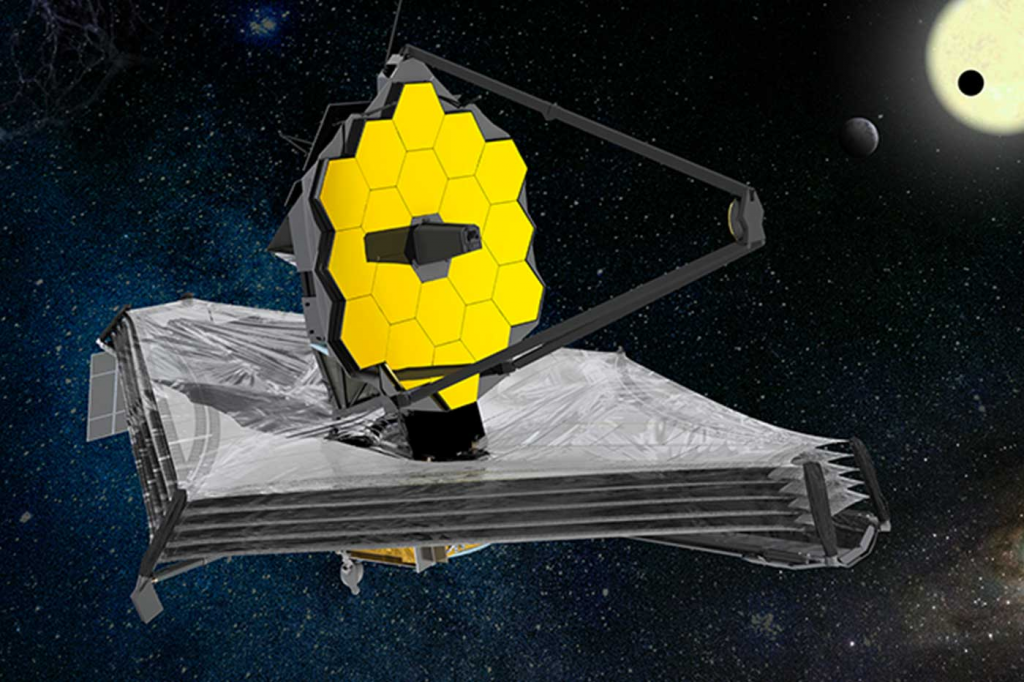
Josh Milliner will always remember the gloomy days of August 2017 when hurricane “Harvey” shattered Texas. As a project scientist for integration, testing and commissioning of the James Webb Telescope (JWST), he could not just hide at home. The giant space telescope, at that time already 10 years behind schedule and considerably over budget, was right in the middle of one of its space-simulating test campaigns at NASA’s Johnson Space Center in Houston. The hurricane had submerged the access gate under several feet of water, and most of the center was off limits. But JWST testing could not stop, the cooling system was still up and running, and there was no way the intense campaign could be further delayed. The final tests inside the 12-metre diameter Chamber-A (built in the ‘60s to test equipment for the Apollo missions) involved lowering the telescope temperature to about 50 K, and create a vacuum similar to that of the deep space. All instruments were tested multiple times, as well as every single, smallest part of the most complex, daring, and expensive instrument ever built to observe the universe. Imagine, working at something that will get to function 30 years later, each piece fabricated and assembled with precision of micrometers, to be shipped at a location in the dark space 1,5 million km away from the Earth. If anything went wrong, the 10-billion dollars enterprise would be dead. With the Hubble Telescope, at 545 km above the Earth, it had been possible to send six different space missions to fix it, during its 30 years of operation. No such thing would be possible at 1,5-million km distance for Webb: failure, simply not contemplated. Everything must function perfectly, and pray no major accident should ever happen, such as a wandering meteorite crushing into the precious 25 square meters of gold-covered beryllium mirrors, whose 18 hexagonal tiles of 1,3m width are constantly realigned by 132 micromotors with ±0,1-micron movements. On Dec. 25, 2021, Webb finally started its travel to the outer space, and reached its destination exactly one month later, Jan. 24, 2022.
As we all learned from Johannes Kepler, the angular velocity ω of an object of negligible mass m, orbiting around the Sun mass M (also the Earth is negligible on this scale) is a pure function of the orbit radius R. For a simple circular orbit, by equating gravity and tangent force you get GMm/R2=mω2R, or R3=GM/ω2, independent on m. To put the Webb telescope far away from the Earth while keeping the same orbital angular velocity of the Earth, so that it stays aligned with the Sun-Earth axis, one has to consider also the added gravity from the Earth. If we rewrite GMm’/r2 + Gmm’/(r-R)2 = m’ω2r, with m’ and r the mass and distance from the Earth of our telescope, we now have a 5-th order equation for r. The five solutions are the famous Lagrangian points, at which the gravity of the two major bodies (Sun and Earth) equilibrate the force on the smaller body. If you plot numerically this equation, and check where it goes through zero, you will find three spots at which the combined gravitational pull of Sun and Earth keeps the small body in a fixed orbit coaxial with the Sun-Earth direction (the other two solutions are placed along the Earth’s orbit, at ±60 degrees). The spot at r=1,51 million km away from the Earth (four times the Moon’s distance) is the Lagrangian L2 point. Because of its mechanical stability that’s a very crowded region of space with other satellites sitting there, like Planck, Herschel, WMAP. However, all the three spots along the Sun-Earth axis can become unstable along the radial direction, hence Webb requires small orbital corrections to keep its position about L2 and stay in synch with the Earth’s motion.
The telescope is not sitting exactly at the L2 point, otherwise it would be permanently under the shadow of the Earth, but makes a wide orbit in the plane perpendicular to the Sun-Earth axis, centered at L2. In this way it captures solar energy to power most of its onboard systems. However, the exposed side of the satellite gets heated by the Sun to high temperatures, which would perturb the functioning of the spectrometers and cameras. The whole apparatus is therefore mounted on top of a huge solar shield, made of five parallel sheets of about 14×21 meters (roughly the size of a tennis court). Each sheet is a layer of Kapton of about 40-micron thickness, covered by aluminum foil on both sides; the two outermost layers also have a silicon coating to increase reflectivity. In normal operation the temperature on the Sun side of the shield may reach up to 400 K, ten times higher than the opposite face holding the main mirror. The five lozenge-shaped sheets were clearly too big to be carried in the Ariane rocket, so they were folded in a complex pattern, to be deployed upon arrival at L2. The unfolding was tested many times in the lab since, of the 344 weak spots identified by design, more than 80% had to do with the deployment. And unfolding in absence of gravity could have provided more surprises… which fortunately was not the case.
While orbiting about L2, Webb accumulates angular momentum, which must be dumped somewhere. This is something that I find quite funny. What happens is that during science observations the telescope is pointed at a target in a generic orientation, very rarely aligned with its center of mass. Thus, as solar photons hit the large sunshield, they impose a torque on the observatory. The attitude-control subsystem counteracts this torque by changing the spin rate of six “reaction wheels”, which progressively store excess angular momentum. Reaction wheels (RW) are extremely accurate systems enabling precise orientation of a satellite. These are electrically powered flywheels, arranged at 90 or 60 degrees from each other, a few tens of cm in diameter and few hundred grams in weight. As soon as the rotation of the spacecraft changes, the RW trigger a counter-rotation in same ratio, through conservation of angular momentum. If a RW reaches this top speed (typically about 5,000 rpm) the spacecraft loses control authority over that wheel’s axis, unless the wheel can be slowed down. Of course, simply applying some kind of brakes to the wheel cannot work, because the momentum subtracted would be released into the spacecraft, causing it to spin back. One solution is to simultaneously slow the wheel down and fire the thrusters, to provide an antisymmetric torque. So, the spacecraft maintains its attitude by transferring the angular momentum stored in the reaction wheel, into the propellant mass, which is expelled by the spacecraft. This is why these events are referred to as “momentum unloads”, or “momentum dumping”. Each momentum unload activity on Webb takes a few hours. The observatory slews to a particular orientation, to minimize the impact on the orbit, then fires thrusters for a few seconds, to allow the spin rates of the reaction wheels to be adjusted.
As far as the astronomical, astrophysical and cosmological results of the first year of operation, you readers can have a look at the website https://webbtelescope.org/resource-gallery/images and see for yourself, I promise you’ll be amazed. For early collections of exciting discoveries, you may check e.g. https://www.nature.com/articles/d41586-022-01931-5, and https://www.nature.com/articles/d41586-022-02056-5 . Here I am rather pointing at some technicalities of this extraordinary laboratory, which were detailed in a very recent preprint of Jan 3, 2023 ( https://arxiv.org/abs/2207.05632 ). From this report, it appears that up to now Webb is functioning perfectly, going even beyond the specifications. Webb’s detectors work between 600nm and 28 microns, that is from orange-red to the deep-IR. The reason for such a choice is that Webb is designed to observe the farmost astronomical objects. Because of the expansion of the universe, the older an object, the larger its displacement to the “red” side of the electromagnetic spectrum. The redshift zmeasures the variation in wavelength due to the apparent recession of the light source from our observation point, z=Δλ/λ. By using Hubble’s law, z=H0D/c, the distance D of the light source can be estimated. Then, by assuming some model of the universe, the light-travel time tL can also be estimated; subtract this value from the (currently accepted) age of the universe, T=13,72 billion years, and you get the presumed age of the light source (star, galaxy, gas cloud…). The Hubble telescope had measured the largest value z~11 for the galaxy GN-Z11, that we see now as it appeared 400 million years after the Big Bang. With its IR instruments, Webb is supposed to be able to show galaxies up to z~20, that is stuff produced just 150 million years after the Big Bang. At z~20 a star like the Sun, whose spectrum peaks around 500nm, would appear peaked around 10 microns, hence the need to go to IR to observe such distant and old galaxies.
From the arXiv report, we gather that all instruments are now working at the best of their capabilities. The two cameras NIRcam (for near-infrared) and MIRI (for medium-IR) are presently operating with performances largely surpassing the design requirements, with a measured RMS wavefront error less than half the predicted value. The Fine Guidance System (FGS, a kind of GPS for orienting the telescope in the space) is so precise that its imaging system has even discovered that some of the reference ‘stars’ in its catalog are in fact galaxies. FGS is coupled to a wide-angle spectrometer NIRISS. The NIRcam is cooled at 35K and covers a field of 2 arc-minutes (equivalent to a square of 1 mm2 placed at 2m distance) with nothing less than 10 independent, 4-megapixel CCDs, each image of a composite field requiring about 10-12 hours of exposure during which the orientation of the telescope must be stable at best than 8 arc-seconds. Yet, a single field of NIRcam may contain hundreds of galaxies, so to get individual spectra from each object, the NIRSPec spectrometer is designed with a system of masks and micro-shutters. NIRcam is also used to periodically realign the 18 hexagonal segments in the whole main mirror. The MIRI subsystem (camera plus spectrometer) works on the medium infrared (5 to 28 microns) therefore it has to be cooled to even lower temperature of 6K, with a closed liquid-He circuit. All the image data gathered are sent to Earth, with just a minimum on-board processing (a DAC converter that absorbs just 11 mW), at a rate of 28 Mbit/s, and are constantly collected by the NASA Deep Space Network.
The Webb telescope is predicted to remain in operation for many years from now. It is powered by solar panels, whose performance could in practice be degraded only by cosmic radiation. All its cooling systems are closed-circuit, in theory with unlimited lifetime. One limiting factor is the amount of fuel used by the thrusters, for orienting and repositioning. At the current work rate, Webb fires the thrusters for about 10 seconds every three weeks. At launch, 200 liters of hydrazine were loaded, that could ensure a maximum flight time of 10 years. However, the trajectory and initial positioning at L2 was so precise that Webb used almost no fuel, so the lifespan could in principle reach up to 20 years. The main threat now comes from micrometeorites. Theoretical estimates gave a value of about one collision per month with objects of 1 gram or less, and indeed in the first six months five impacts were recorded. However, the third impact in May 2022 was stronger than imagined, and led to an unrecoverable loss of optical performance, by about 4%. Given the current above-standard performances of the telescope, this was not a major problem. However, future impacts could accumulate, and slowly but inexorably degrade the fantastic quality of the images that we saw in this first year.
Within days of the first Webb data release in July, non-peer-reviewed papers began flooding the arXiv preprint server, reporting multiple candidates for the most distant galaxy ever recorded, and lots of hypotheses about “new visions” of the early universe. In September, however, a recalibration of the instruments (notably NIRcam) forced many astronomers to rethink some of their early findings. It looks like somebody rushed ahead of themselves, in the initial wave of excitement. However, we are just at the beginning of 20-plus years of work, and it’s easy to predict a lot of changes ahead. “Right now I find myself lying awake at three in the morning,” says Jane Morrison, University of Arizona and member of the JWST team, “wondering if everything we’ve ever learned until now is wrong.”