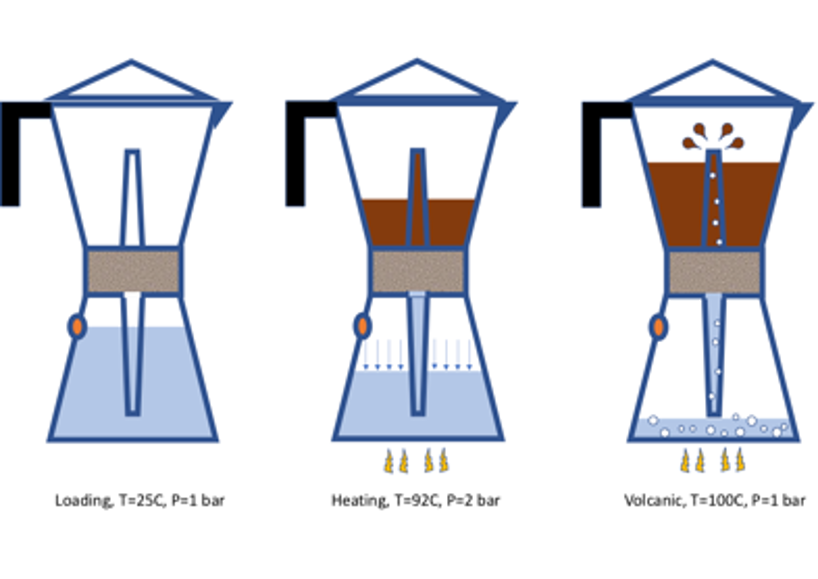
When I first arrived in Argonne, more than a quarter of century ago, I was a visiting professor, and obviously (noblesse oblige…) I could not sit in the communal students’ rooms. Short of individual offices, the only solution was to place my 66-Mhz PowerMac 7100 in a large spare room, which also doubled as coffee facility. Two huge percolators were sitting on the table next to mine, and people used to come in at any time. The coffee was definitely awful (a percolator brews continuously at near-boiling temperature, and the coffee gets increasingly bitter and burned), but many Americans love their coffee well-brewed and very watery. The problem was that each person that entered the room considered a duty to speak some words of politesse to me, and as a result I could not concentrate on work for more than 5 minutes without some interruption. However, since Americans seem to love air conditioning even more than coffee, the solution I found was to set the room temperature to Italian summer levels (that is, above 100F): after which, people started to quickly fill their coffee mugs and run away from the torrid temperature, without interfering any longer. I never liked that kind of coffee, although I do appreciate a good filter coffee or a french press.
One may think that the complexity of coffee-making should lie in the secrets of chemistry, and that may well be. However, as far as the major differences, the main is a physical one between brewed and espresso-like coffee, and it is pressure. All types of brewing, dripping, pouring, or percolating machines (including the very popular “dosettes”) work at ambient pressure of 1 bar. Therefore, the coffee is obtained by gravity-driven percolation of hot water from above a (paper, plastic or metal) coffee holder, which has the only function of keeping the coffee powder under the water flow. Pressure-operated machines, instead, force the flow of hot water at a pressure well above 1 bar, against a mechanical filter: this procedure is meant to improve the dissolution of flavours (essential oils) in a quicker run, increasing about fivefold the fraction of coffee extracts per water mass. A traditional moka pot works at 2-3 bar, a professional steamer keeps a constant pressure of 9 bar, and most home machines (e.g. Nespresso, Krups, Delonghi) declare a peak pressure of 15 bar. For comparison, tires in your car are at about 2 bars, a steam-iron works at about 5 bars, and the Space Shuttle hydrogen tank was at 4,4 bar.
The moka pot is a simple, three-parts aluminum device (see my sketch enclosed), invented in 1933, which despite its simplicity contains a lot of physics. Most people think that the heat brings water to boiling, and this flows up in the filter to make the coffee. However, its functioning is much more subtle [ see: C. Gianino, Am. J. Phys. 75, 43 (2007) ]. The key is actually the amount of air stored above the water (at the level of the control valve, left sketch): heating warms both the water and the air, starting a thermodynamic cycle. Air mixes with water vapour and is compressed by increasing temperature, never going above 90-92C; upon reaching this temperature, the gas has expanded enough to develop a pressure of about 2-2,5 bars (as regulated by the valve, adjusted to match the filtration pressure opposed by the coffee powder… ehm… try to press your coffee too tight in the filter, and see what happens!); this pushes (isobaric expansion) the hot water up the funnel and homogeneously wets through the coffee powder: now, a coffee-water solution starts to flow gently from the upper tip of the funnel (center sketch). But once the inner water level drops below the lower tip of the funnel (right sketch), pressure instantly drops at constant volume down to ambient, since the above tip is open, and water temperature jumps to the boiling point: residual coffee spurts at the tip of the funnel like a small volcano. At this moment, you have to stop the fire, and move the moka away from the stove, to avoid boiling water to keep flowing and ruining your coffee into a bitter and much acidic solution. (Note: to close the thermodynamic cycle you have to refill, at ambient P and T…)
The ideal values of about 92C temperature and 9 bar pressure for an optimal extraction of essential oils from coffee powder have been established long time ago. Professional machines, as found in the best coffee shops (https://www.roastycoffee.com/commercial-espresso-machine/), are designed with a mechanical barostat to keep the value of 9 bar reasonably constant for the whole time (and on more than one serving line in parallel). But then, why Nespresso & co. advertise 15-bar machines? If 9 is good, does a higher pressure make an even better espresso ? Again, a little physics helps to understand. The pitfall is that a home machine is much less sophisticated, and is not capable of maintaining a constant pressure throughout. Darcy’s law of filtration states that the pressure drop is given by 𝛥P=𝜂LQ/k, i.e. proportional to the product of fluid viscosity 𝜂, tube length L and volumetric flow Q, and inversely proportional to the filter (i.e., coffee’s) permeability coefficient k. Of these quantities, the latter is the one that changes more drastically, decreasing by about a factor of 10 going from dry to fully wet coffee powder [ see: L. Navarini et al, Appl. Therm. Eng. 29, 998 (2009) ], while Q increases from zero to some positive value. Therefore, the pressure in such a machine increases steadily during the infusion process: 15 bar is just the peak at the end of the process, while an average of about 9 bar should (hopefully) be in effect.
The next step for coffee aficionado is mixing with milk, which results in a wide range of combinations ranging from latte (caffelatte or café-au-lait) to cappuccino, and many more. In this case, yet without knowing, the barista is exploiting the elegant physics of double-diffusive convection. DDC occurs when two fluids of different density, temperature and diffusion rates are mixed dynamically. Although it was introduced by Melvin Stern only in the early ’60s to describe oceanic “salt fingers” – the thin, alternating fluid filaments that appear when warm and salty water overlies fresh and cold – the theory of DDC rapidly found wide applications in astrophysics, geophysics, chemistry and materials science. DDC is driven by the difference in the molecular diffusivities of heat vs. solutes, and operates in a counterintuitive way: it is a mixing process that makes the dense fluid denser, and the light fluid lighter. The most dramatic signature of DDC is observed in the stepped structures of vertical temperature and salinity profiles in the oceans (the thermohaline staircases), which can also be routinely reproduced in the laboratory. Yet, many questions about the detailed origin of the phenomenon go unanswered. Recently, a group of physicists and engineers exploited DDC to explain pattern formation in café-au-lait and multilayered gels [ see: Laboratory layered latte, Nature Comm. 8, 1960 (2017) ]
Distinct horizontal layers may form when pouring espresso into a glass of warm milk (see their Figure 1c,d), a phenomenon that can be randomly observed also when you make latte at home. The act of pouring, forces a lower-density hot liquid (coffee) into a higher-density ambient (milk). The downward inertia caused by pouring is opposed by the buoyancy of coffee, with a dynamics similar to the fountain effect (also characteristic of injecting fluids into a second phase at different density). The interesting discovery from these experiments is that the formation of layered structures at different densities depends on the injection speed. The injected fluid dynamics appears to establish circulation cells in planes perpendicular to the vertical density gradient, with a radial temperature gradient from the center to the boundary of the container. Layering is observed above a critical injection velocity, resulting in the competition between the thermal cooling and the density gradient, and quantitatively characterized by the Rayleigh number, Ra = (ga∆T/nk) (a∆T/∆r’)3, with g=9.81 m/s2, ∆T the temperature gradient, ∆r’ the normalized density gradient (represented by salt concentration, in the experiments), a the thermal expansion coefficient, n the kinematic viscosity, and k the thermal diffusivity of the injected fluid (that is, coffee). The critical injection velocity corresponds to a critical Rayleigh number of about Ra~10,000, above which the nice layers of coffee and milk mixed in different proportions can happily sit on top of each other (and it may be useful as well to prepare multi-layered soft gels for e.g. biomedical applications).
Besides its paramount importance in obtaining a fancy cappuccino, the physics of DDC comes into play in complex systems in which a thermal and composition gradient interact. This could be also the case of nanofluids, proposed years ago for heat transfer applications. As you may know, there is still a substantial controversy in this field, since theoretical models invariably predict exceptional improvements in heat conductivity of the fluid upon addition of nanoparticles, whereas experiments have given conflicting and sometimes inexplicable results. Whether DDC provides the most proper explanation, or heat transport is classical (meaning it does not require any special “nano” arguments to be explained), the final word seems not be written yet.
And now I am going to make my tea, and happy coffees to everybody.