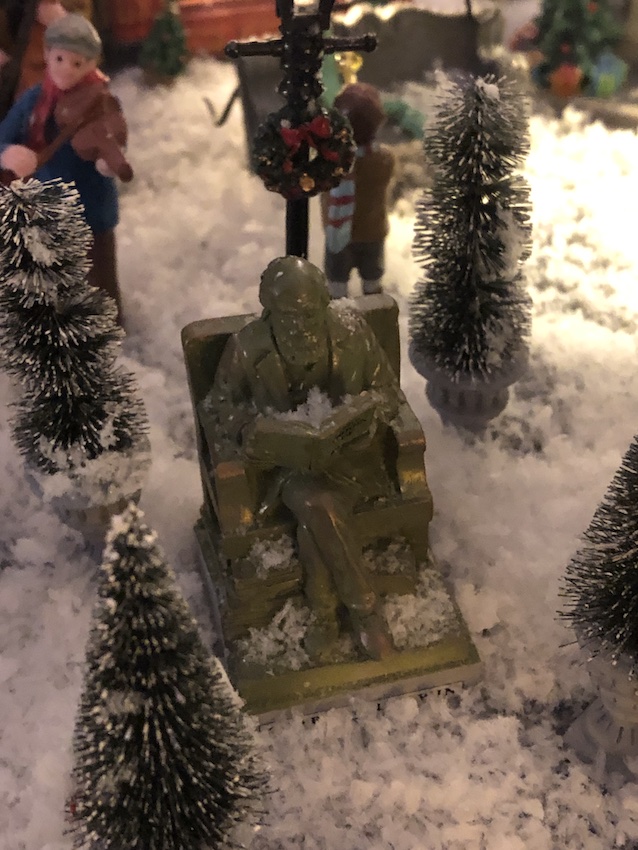
Today is New Year’s Day, according to the old Julian calendar. After celebrating yesterday night, with a magnificent all-Russian menu (including shuba, two variants of Olivier, lots of kilka and sprats, and French champagne, and rewatching again the classic 1975 movie Ironyia Sudby), it is time for Olga and me to start removing all Christmas-time decorations from the house. These include a large winter, Victorian-themed village, with its main square hosting a little statue of Charles Darwin (photo enclosed), who in some way is the main character of today’s story. That’s about evolution, and its maybe most striking outcome, that is human intelligence, and its maybe most unexpected outcome, that is artificial intelligence.
We often come across statements from people criticizing science, like “I can’t believe that something as beautiful and complex as the human eye could be the result of a random process like evolution”, or “It seems implausible that the intricate molecular machinery of the cell, such a finely-tuned nanofactory of exquisite complexity, could have arisen by chance”. The basic argument being made is as follows: 1. Highly complex, organized, functional parts of the body, the brain, the cell’s inner workings, could not possibly have arisen by chance. 2. Evolution is a chance process. Conclusion: complex parts of animal body and brain cannot be a product of evolution. The fatal flaw in this argument is that premise 2 is incorrect. Evolution is not a chance-driven process, this is just a widespread misconception.
To see why, we can break evolution down into two steps. Step 1, Mutation. This step introduces new genetic variants into the population, and is indeed random. Mutations do not arise in order to fill a current “need” of the organism. They are blind, and have no foresight, so they also cannot anticipate future needs. In this sense, they can be described as random, also in the sense that they are not automatically helpful: a new mutation may turn out to be beneficial, or harmful, or neutral altogether. Step 2 is Natural selection: some of the new, genetic mutated variants can make it into the next generation, and some do not. (This is a bit of a simplification, but it is good enough for our current purposes). However, this second step of natural selection is not random at all. In fact, it is the exact opposite of randomness. Mutations that turn out to be beneficial to the organism are more likely to make it into the next generation, precisely because they help the organism’s survival, reproduction, or social success. Mutations that are harmful are less likely to make it into the next generation, precisely because they lower the organism’s likelihood of survival and reproduction. Natural selection is, apart from fluctuations due to a number of environmental constraints, logical and predictable: the likelihood that a mutation will make it into the next generation depends, in a rather predictable way, on its effects on survival and reproduction. Beneficial mutations tend to get passed on, whereas detrimental ones are weeded out. The mistake is that people sometimes confuse mutations (which are random) with natural selection (which is not random), taking evolution as a whole as an entirely random process.
Nevertheless, among scientists the debate on which step should be most crucial to the evolution, namely the random seeding of mutations vs. the systematic statistical selection of the most “useful” mutations, has been (and is) an active subject of discussion. The greatly missed Stephen Jay Gould was famous for describing the evolution of humans and other conscious beings as a “chance accident of history”. If we could go back millions of years, he used to say, and run the tape of life again, evolution would likely follow a different path. A good example is provided by the octopus’ brain system. As Peter Godfrey-Smith suggests in his beautiful book Other Minds, the octopus is the closest we will ever come to meeting an intelligent alien. Our last common ancestor goes back some 600 million years. Hence the mammalian side of the tree of life, evolving from our common ancestor, slowly developed into beings of highly complex intelligence (that is us, although when watching the daily news, we should doubt it). On the other side of the tree, another line developed into a whole bunch of other creatures with limited brain capacity (such as the various invertebrates), except for the Cephalopods, who are much clever, with the octopus at the top of the ladder. These bright, cheeky, curious creatures and their intelligence developed independently and separately from our side of the tree, which is a clear example of how evolution could take very different paths, according to just some random mutations occured in the common ancestor that caused the split, and ending up with rather different bodies (two legs and two arms instead of eight tentacles) and rather different modes of “intelligence”.
When one moves at the molecular scale, however, randomness seems to hold the primacy. A few years ago, a study by University of Pennsylvania biologists claimed to provide evidence that evolution is both unpredictable and irreversible, when looked at the smallest scale relevant to biology. Using computer simulations of an evolving protein over a fictitious time span corresponding to 10 million years of random evolutionary mutations, they showed that the genetic mutations that come to be accepted by evolution are typically dependent on mutations that came before, and the mutations that are accepted become increasingly difficult to reverse, as time goes on. Notably, their study focused exclusively on the type of evolution known as “purifying selection”, which favors mutations that have none, or just a small effect in a given environment. This is in contrast to adaptation, in which mutations are selected if they increase an organism’s fitness in the environment. The result that later mutations are dependent on the earlier ones demonstrates a feature known as contingency: mutations that are accepted by evolution are contingent upon previous mutations to ameliorate their effects. The team then asked a distinct, related question: whether is it possible to revert an earlier mutation, and still maintain the protein’s stability. The answer was no: mutations became entrenched and increasingly difficult to revert as time goes on. While random substitutions are made at each point in time, no major change in protein stability are observed. However, after a certain number of mutations, going back and trying to revert one earlier change the protein structure begins to collapse. Both contingency and entrenchment are known to be characteristic of adaptive evolution, in which selected mutations improve the fitness, that is they are not simply additive changes, but enrich one another (epistasis); however, it appeared as a surprise to observe these effects also in purifying selection that, by definition, should involve only ineffective, neutral mutations. The overall result would be that predicting evolution on molecular basis is impossible, since on this scale adaptive mutations are not distinguishable from neutral ones, and randomness seems to dominate.
However, such a reductionistic view of protein function could not stand a more global perspective. Molecules in the cell are not just casually passing by and popping in some function, in a mitochondrion or on a piece of DNA. They evolved and were (spontaneously) selected according to increasingly complex needs, and operate in close cooperation, according to rather tight scheduling, to maintain vital cell functions. We know for example that protein 3D-folded structure is much better conserved than protein sequence (the list of amino acids that makes up the protein). By looking with x-ray diffraction at the 3D shape of the folded protein hemoglobin, for example, it can be observed that there are but very minor variations in shape between the human hemoglobin, and that of an elephant, or red tuna, or a pigeon. However, when we analyse the amino acid sequence of these proteins, which perform exactly the same function of carrying oxygen in the blood in those different animals and apparently share a very similar folded shape, we find that only 80% of the elephant hemoglobin resembles the human, and this fraction drops to 45% in the red tuna, and to a striking 25% sequence identity in the pigeon. The reason, as we currently understand it, is coevolution: when two amino acids are found in close contact in the 3D structure, a random sequence mutation in one (e.g., by a UV-ray striking the DNA fragment coding for it) must be closely followed by a comparable mutation in the other one. If this does not happen, the first mutation is discarded, since the protein 3D shape would be too much altered to function properly.
A more recent study, just published in PNAS, challenges the long-standing belief about the unpredictability of evolution at the molecular scale, and has found that the evolutionary trajectory of a genome may be influenced by its entire evolutionary history, rather than determined by random factors and historical accidents. The team at University of Nottingham used artificial intelligence tools, to carry out an analysis of the pangenome, that is the complete set of genes within a given species. Using the “Random Forest” machine-learning approach on a data base of 2,500 complete genomes, all sequenced from a single bacterial species, they firstly created “gene families”, in order to compare like-with-like across the genomes. Once the families were identified, they analyzed the patterns of how these families were present in some genomes, and absent in others. They found that some gene families never turned up in one genome when a particular other gene family was already there; and on other occasions, some genes were very much dependent on a different gene family being present. In fact, they discovered an invisible ecosystem where genes can cooperate, or can be in conflict with one another. Such interactions between genes make at least some aspects of molecular evolution somewhat predictable, and furthermore, the AI-based tool now allows to make those predictions. The implications of this research could be “nothing short of revolutionary”, in the words of James McInerney, the lead author of the study. The demonstration that evolution at the molecular scale is not as random as usually thought, opens the door to an array of possibilities in synthetic biology, medicine, or environmental sciences, for example providing a roadmap for the predictable manipulation of genetic material, fighting antibiotic resistance, targeted and personalized treatments, and many other medical applications.
The dilemma of randomness vs predictability is not an easy one to solve, and it continues to spur both scientific and philosophic controversy, likely as a reflection of the growing influence (especially in the USA) of the so-called “intelligent design”, a pseudo-theory that combines the worst of all possible worlds, being at the same time non-science and flawed theology. However, think that Darwin himself predicted in 1862 the discovery of as yet unknown species of moth, based purely on the length and shape of a specific orchid flower spur found in Madagascar, which in some instances can be over 25 cm in length. Darwin wrote in a letter to a friend: “I have just received such a box full from mr Bateman with the astounding Angraecum sesquipedalia with a nectary a foot long. Good Heavens what insect can suck it. In Madagascar there must be moths with probosces capable of extension to a length of between ten and eleven inches.” Darwin’s prediction was verified only 130 years later.